Instructions for making the Sun: How the race for nuclear fusion has accelerated
Driven by the latest scientific advances and a huge wave of investment in private projects, the dream of generating energy by replicating the processes that keep the stars alight is no longer science fiction
The impact of 192 powerful ultraviolet lasers on a tiny capsule just two millimeters in diameter occurred a few minutes after 1 a.m. It was December 5, 2022, and the group of scientists directing the experiment from the control room at the Lawrence Livermore National Laboratory in California burst into celebratory applause when they saw the results on the screens: they had achieved 3 megajoules of energy by using lasers to shoot 2.3 megajoules of energy at a small fuel pellet. After decades of research and hundreds of millions of dollars in investment, obtaining the energy equivalent to that needed to heat water for a shower may not seem like much, but they had actually just demonstrated that it is possible to create energy by reproducing on Earth the physical reaction that powers the Sun and all the stars in the sky.
For more than 70 years, humanity has been pursuing the dream of turning nuclear fusion (when the nuclei of two atoms fuse together, the opposite of fission, which separates them) into a source of energy that is expected to be safe, clean (it creates waste, but it is very manageable and it does not emit greenhouse gases) and almost inexhaustible: its main fuels are easily found in nature (for example, seawater as a source of deuterium atoms). But until that December night, when that tiny pellet exploded in the center of a vacuum chamber in California, no one had managed to generate a positive result, that is, produce more energy than was actually used to join the atoms.
“It has been a turning point, it has given the world confidence that it is possible. It’s been like the Wright brothers’ experience [who in 1903 made the first controlled powered flight],” says Bruno Van Wonterghem, head of operations at the National Ignition Facility (NIF), the center of the large public complex in Livermore, California that is carrying out the fusion project.
In the lobby of the same building one morning last June, amid reproductions of laser optics, display cases showing key pieces of work such as fuel capsules, and a few exhibits celebrating the lab’s records, Van Wonterghem continued his analogy. “It’s like we’ve gotten the plane off the ground, now we have to see how high we can go, how fast we can fly… We’re moving very fast; we’ve gone from 3 megajoules of output to 2.2 megajoules of laser energy to 5 [in later experiments] and we hope to get up to 10 this year. And then with some small improvements to the laser, to 30 megajoules, which is a gain of 10 or more [relative to incoming energy].”
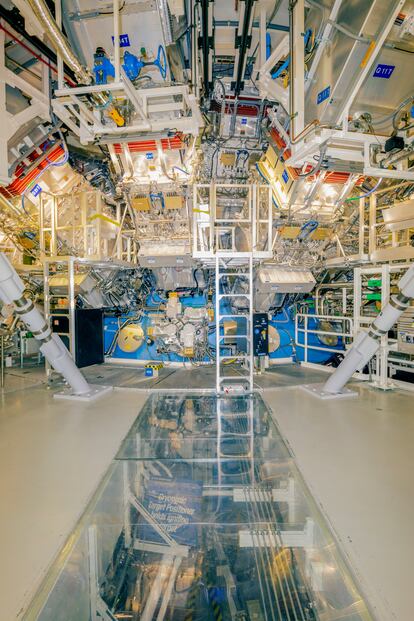
The truth is that to achieve a viable power plant with lasers, a gain of between 50 and 100 times, relative to the energy input, would be needed. And not only that, it would also need to fire at least 10 shots per second (at the NIF, one shot every seven or eight hours is currently possible) and with much more efficient lasers (the NIF lasers require an enormous amount of energy to start the whole mechanism). And the machine in the Livermore National Laboratory will never achieve this, to begin with, because it is not made for that; it is an experimental scientific facility primarily aimed at studying nuclear weapons. Other labs will have to find technological solutions to engineering challenges that seem like science fiction, since they consist of creating a power plant that reproduces on Earth the same processes that occur in the Sun at millions of degrees celsius and with a pressure equivalent to 100 billion Earth atmospheres.
In fact, the research path being pursued by the Livermore laboratory — inertial confinement (achieving fusion based mainly on pressure) — has always been seen to have less potential than magnetic confinement, which seems to have the best chance of reaching the goal, although it still has its own problems to resolve. Magnetic confinement consists of exposing atoms to such high temperatures (more than 10 times the temperature of the Sun) until the nuclei overcome their natural repulsion and have no other choice but to fuse. But no one doubts that the demonstration by the Californian laboratory is at the heart of the advances that have revived the race, which had been stalled for decades.
Germany has launched a €1 billion ($1.11 billion) research plan through 2028 that integrates both magnetic and inertial pathways for the first time. Its goal is to have a fusion power plant ready by 2040. The United Kingdom wants to have its own STEP plant ready in the same decade, to be built on a former coal-fired power station in West Burton, central England. China, which spends around $1.5 billion a year on the sector, plans to have its first industrial prototype of a fusion reactor, dubbed the “artificial sun,” by 2035 and begin large-scale commercial production by 2050. The U.S. Congress approved a record investment of $1.48 billion this year.
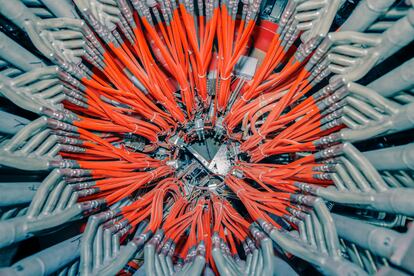
“Right now, I’m having a hard time finding enough PhD students and postdocs to work on all the projects I already have funding for. And that’s happening on a global scale. We have funding to tackle the enormous challenges we have ahead of us, but we don’t have enough of a workforce to do it,” says Jaime Marian, a professor at the University of California, Los Angeles (UCLA) and an expert on materials suitable for fusion reactors. The scientist believes that, in reality, public investment, at least in the United States, has been trailing behind a private sector that sees nuclear fusion not only as viable, but potentially very lucrative.
This year, the Fusion Industry Association surveyed more than 45 companies (25 of them in the United States), with a total of $7.1 billion in funding; in 2020, when it published its first report, it surveyed 25 companies, with $1.9 billion. Most of the money comes from private investors — including Jeff Bezos, Bill Gates and companies like Google — but public investment has risen from $85 million to $426 million.
“We recently received a significant grant, 50% funded by the British government and 50% by us. We also work with Imperial College, Oxford University and the York Institute of Plasma Physics. It is a £12 million [around $16 million] research program over five years,” explained Nick Hawker, founder of First Light Fusion, at the company’s headquarters in Oxford earlier this year.
The company, founded in 2011, is trying to achieve the same goal as Livermore, but instead of using a laser, it uses projectiles launched from a powerful 22-meter-long cannon. The key to achieving the same effect as a laser is an amplifier for the fuel capsule that “increases the pressure and also shapes the energy so that, even if we hit, we get a spherical implosion,” explains Hawke. “It is our key technology,” he adds shortly before offering a tour of the laboratory where the cannon is located.
When loaded with three kilos of gunpowder, the projectile launcher — nicknamed the Big Friendly Gun — launches projectiles at 4.4 miles per second. These hit the fuel capsule inside a vacuum chamber similar to the one in Livermore, but smaller: the one in California is 10 meters in diameter and this one is 1.7 meters.
“The engineering of our approach is tremendously simpler, so is the engineering necessary to get to a power plant, too. And it is much cheaper,” says Hawke. The initial design for a pilot plant has already been completed. “Our goal is to achieve this in the 2030s. Just like all private companies. Among public programmes, even the most ambitious ones talk about 2040. I am convinced that a small, agile private company can go faster than these large institutions,” he says.
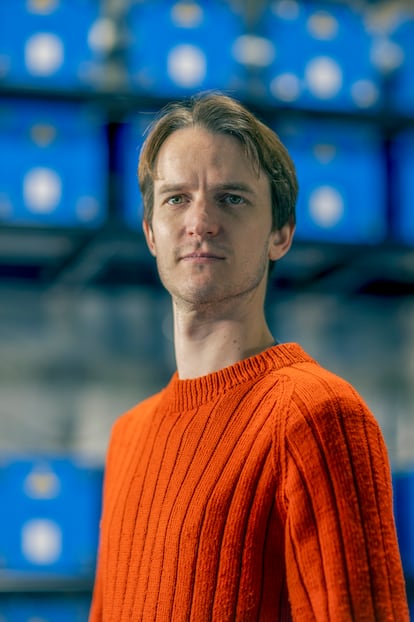
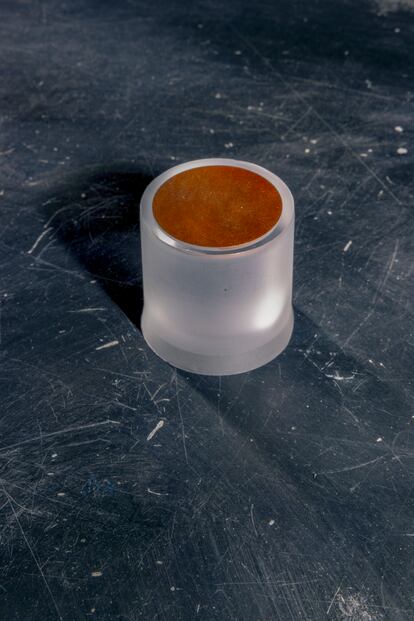
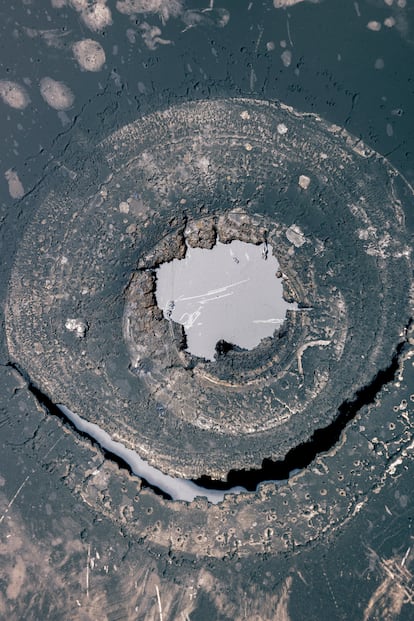

To explain his point, Hawke cites an international laser research project that, when it needed to renew its facilities, had to contract new suppliers from different countries because every one had to get a slice of the pie, which greatly delayed the entire process. In fact, the delays and cost overruns of the gigantic international fusion energy project that has captured almost all the sector’s attention would seem to prove him right, at least in part.
Backed by 35 countries — including the EU, the U.S., Japan, South Korea, China and Russia — the ITER (Latin for “the way”) is an experimental reactor of colossal dimensions, theoretically big enough to prove that it is possible to make viable magnetic confinement power plants. It aims to achieve what is known as the triple product: high temperatures — at least 100 million degrees Celsius — a pressure of more than five atmospheres and an energy loss time (if the gas is no longer heated) of more than three seconds, creating at least 10 times more energy than it needs to run — 500 megawatts from 50 megawatts.
It also includes a set of 1,000-ton magnets capable of magnetically controlling the shape and placement of plasma — the soup that particles become when they reach such high temperatures. Magnets made of superconducting materials that, in order to work, must be kept at very low temperatures. “In just four meters you can have temperatures of around 300 million degrees Celsius at the center of the plasma and less than 200 degrees below zero in the magnets. There you have the Sun and here the dark side of the Moon,” says Alberto Loarte, chief scientist of ITER, standing next to the vacuum chamber where the plasma will be generated, a monster 19.4 meters in diameter and 11.4 meters tall that weighs around 5,200 tonnes.
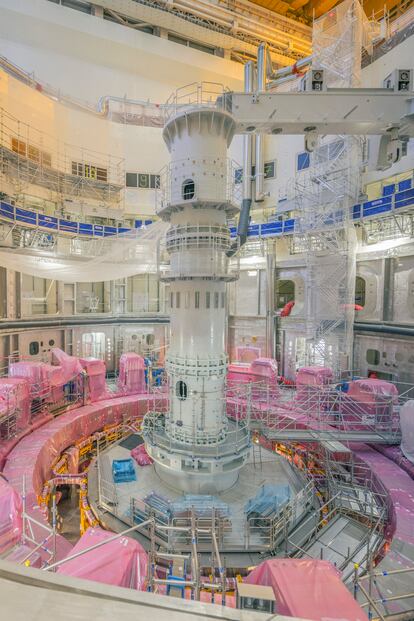
In 2007, when the organization responsible for building the ITER was set up in Cadarache, in the south of France, the goal was to generate reactions that would produce energy by 2025. In the meantime, however, design flaws, management and manufacturing errors, some clashes between security officials and a global pandemic have pushed back that date. This past summer, it was estimated that the first reactions will take place in 2039. From an initial budget of €6 billion ($6.7 billion), it is estimated that the project will end up costing well over €20 billion ($20.26 billion). The plan is for its results to be used to create a plant capable of supplying electricity to the grid. Europe, for example, plans to build its pilot plant (DEMO) in 2050.
The problem is that the rate at which research and technology are advancing elsewhere is accelerating exponentially, so there is a chance that by then, they will have been overtaken by one of the various alternative routes that promise to do the same thing sooner, with smaller, more manageable and therefore more economically viable machines. This is probably why countries are now re-positioning themselves and revising their strategies.
“The others are getting organized. China has a plan, the U.S. too. And I think there is a European initiative too,” says Marc Lachaise, director of Fusion for Energy, the Barcelona-based EU organization responsible for European contributions to ITER. He adds: “We have been collaborating for years and we have done very well, but I think it is time for Europe to create a new roadmap that is adapted to the context and makes sense for everyone.” In any case, he continues: “ITER remains the common goal and it is not just in Europe; it is the largest project that everyone is looking at because no start-up can do something similar.”
In fact, a good part of the scientific community raises a skeptical eyebrow when they hear the promises made by private companies. They admit, however, that a startup could hit the jackpot thanks to their agility, ability to attract talent and disruptive approach.
That is how Sam Davis, project manager for the JT60 experimental reactor in Japan, sees it. One of the little brothers of ITER, which is carrying out tests to pave the way for the French giant, the JT60 is actually achieving some magnificent results that have contributed, along with Livermore, to breathing new life into the sector. For example, the energy record of 69 megajoules achieved a few months ago at the British JET complex.
“For many years, progress has been slow, funding has been quite scarce, and people have been forced to do international collaborations, in part because no country could do it alone. So this wave of private investment is very welcome,” explains Davis. He continues: “Many of these new companies are not going to be able to keep their promises, but they can solve some specific problems. And if you have 10 companies and they all solve a problem, you know, that’s 10 fewer problems that put you closer to the goal.”
But to better understand what problems we are talking about and how close or far away the objective may be, we should go back in history and understand some basic concepts. “What we want to achieve is to reproduce the reactions that take place in the Sun and stars. They work the same way: since they have a lot of mass, gravity forces the nuclei of their particles to fuse to give heavier elements: two hydrogens become one helium. And in this process a lot of energy is released, because a mass deficit is produced, that of Einstein’s famous equation: E=mc² [energy equals mass times the speed of light squared],” explains Isabel García Cortés, a scientist at the Fusion Laboratory of the Centre for Energy, Environmental and Technological Research (CIEMAT), based in Madrid, which has hosted another of these research machines in this field, the TJ-II, since the 1990s. She continues: “The difficulty we have on Earth, obviously, lies in the fact that we do not have the gravity that the Sun has.”
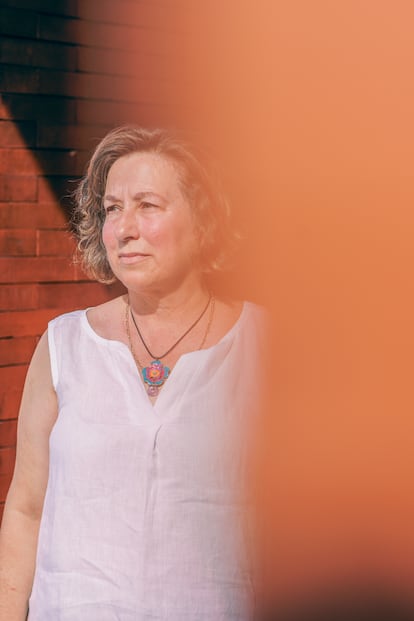
Thus, the two main ways researchers have found to compensate for this and get the two nuclei — which naturally repel each other because they have a positive charge— to fuse together are inertial confinement (by compression) and magnetic confinement (by temperature in a confined plasma for long periods of time). Both require a high vacuum chamber to produce the fusion — when working with hydrogen, the lightest element in nature, any other element can interfere — and the same fuel, which is made up of hydrogen isotopes that are more likely to fuse at a lower temperature.
These isotopes are deuterium — which is easily found in seawater — and tritium, which is not found in nature and must be manufactured, posing a technological and economic problem. When they fuse, the two particles become a single helium particle, and release a neutron charged with a lot of energy. Part of this energy would have to be converted into electricity and another part would be used to produce more tritium (another unsolved challenge). What’s more, these neutrons do terrible damage to any material they hit.
Since its invention in the 1950s, the development that has stood out above all others on the road to trying to solve these issues is a doughnut-shaped machine called Tokamak (the predecessor to ITER), which comes with its own set of problems. The main one is controlling, by means of super-powerful magnets, the kind of soup that particles become when they are very hot (plasma, the fourth state of matter) and confined inside that doughnut, at a temperature and for enough time for fusion to occur that creates an energy gain.
This is precisely the triple gain product that ITER is trying to demonstrate. How? To start with, by volume, with the largest magnets to create the largest plasma in the world: plasma of 840 cubic meters in a flow of 6.2 meters in diameter. The aim is for the plasma, when the time comes, to create by itself most of the energy and part of the magnetic field that it needs to stay hot.
In other words, the plasma will be continuously active to receive the fuel pellets. These will be cryogenized so that they can enter the plasma when they are launched at full speed. Lithium plates (test blankets), probably liquid, will be responsible for collecting part of the neutrons to efficiently convert them into tritium and electricity. And a water-cooled tungsten structure on the floor of the vacuum chamber will extract heat and ash produced by the fusion reaction, minimizing plasma contamination and protecting the walls from high temperatures and neutron impacts.
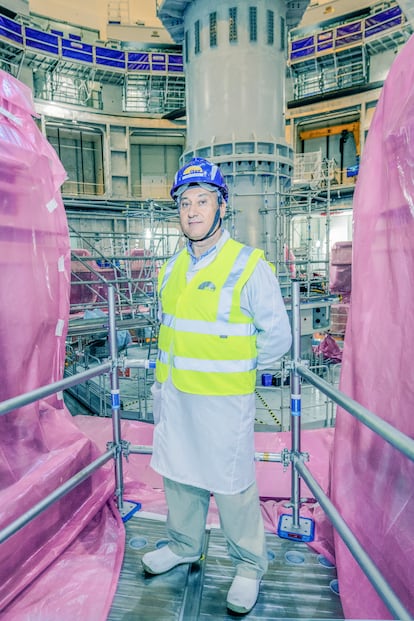
The process is enormously complex due to the infinite number of variables that come into play — from the size and composition of the pellet to the exact shape of the plasma, how quickly or slowly it is heated— and the highly unpredictable scenarios — often it’s not known how a material will react when it reaches a certain temperature or pressure or how that will affect other components, taking into account that they all have to work perfectly at the same time — explains Loarte.
The scientist also speaks of the logical bureaucratic complications of such a large project with so many countries involved, where the idea is that all knowledge is shared. He argues that ITER continues to be leading the way: “I believe that these private companies leave many things open. If you want to show that you have a gas that heats itself and only that, or that I have a gain of five times the energy I put in, you don’t build ITER. But when you want to demonstrate everything necessary for energy production at the same time, I think it is an inevitable step. That is why the United States, which abandoned the project in 1998, returned in 2003. In any case, if someone shows that it can be done faster, that’s great.”
Companies such as the U.S. Commonwealth Fusion Systems and Britain’s Tokamak Energy are working on this, using high-temperature superconducting (HTS) magnets that allow for much smaller and more manageable reactors. The former is already building in Devens, Massachusetts, what its scientific director and co-founder Brandon Sorbom defines as “the world’s first commercially relevant fusion device.” It uses technology that, on paper, can achieve a fusion gain of 10, he says. For now, the goal is to create as much energy as they put in by 2026 and then move on to the construction of a second power plant with which they hope to start supplying electricity to the grid in the 2030s.
Tokamak, on the other hand, has the same objective, but has not yet begun building its prototype. In its case, in addition to the HTS, they are working with a different reactor shape — a sphere instead of a donut. “It is more efficient, and requires a lower capital investment and operating costs and a smaller space,” explains the company’s CEO, Warrick Matthew, via video, pointing out that the state-funded project in the United Kingdom also uses a spherical tokamak.
Companies often approach nuclear fusion by taking up avenues that were long ago abandoned and applying new scientific and technical advances. For example, the Californian company TAE Technologies has designed a machine that takes up the old idea of field reversed configuration (FRC). This creates a toroidal plasma that will theoretically allow high enough temperatures to replace deuterium and tritium fuel with hydrogen and boron. This would avoid the emission of neutrons and, consequently, the damage they cause to materials and the issue of their radioactive load, which is not much, similar to that of accelerators used in hospitals.
Sweden’s Novatron, meanwhile, claims to have found a way to solve the problems that plagued research into “mirror machines,” in which a more powerful and controllable “sausage-shaped” plasma will provide access to a simpler, more efficient and cheaper technology. That’s according to Erik Odén, the company’s executive chairman. Odén says this will also make it possible to work with other fuel alternatives. Novatron’s proposal attracted a lot of interest during a presentation at a meeting of companies in the sector held recently at the ITER headquarters. Its idea has been described by the renowned Berkeley professor Kenneth Fowler as “the missing link in nuclear fusion.”
The proposals are endless. Last year, according to the International Atomic Energy Agency’s count, there were 100 experimental nuclear fusion devices in operation (90 of them publicly funded), another 14 under construction (five of them private) and another 44 planned (more than two-thirds, in this case, private).

The possibilities are so wide and there is so much excitement that it can be difficult to decide what project to back. “What is the best way to use the money so that we are not all doing the same thing? Making sure that we are also addressing the big problems,” says Tammy Ma, who is in charge of the inertial fusion energy initiative in Livermore at the NIF facilities.
Ma has been tasked with proposing a national program, with a view to involving other countries, to market fusion energy. “So with the public program, what we are trying to do is fund the basic technologies that can be used in all approaches. For example, if you need to create tritium, everyone will need the blankets to do it. So the U.S. program is trying to put money in the areas that will bring the greatest benefit to everyone,” she says.
“In all projects, whether it be Tokamak or another one, there is a problem with the issue of materials,” says José Aguillar, coordinator at the University of Granada of the Technical Office for IFMIF-DONES, the ITER program project to build a large particle accelerator in southern Spain that will be able to test the resistance of components before using them in reactors. With construction soon to begin, the IFMIF-DONES is a kind of neutron cannon, unique in the world, which will be fired at materials to predict how they will withstand nuclear fusion. They hope to begin operating at full capacity in 2034. “It will be a scientific infrastructure that will be working 24 hours a day, we will try to make it work every day of the year,” says Aguilar.
With thousands of scientists working in universities and research centers around the world on each of the problems that remain to be solved, the pursuit for nuclear fusion is a strange story of both competition and collaboration. A race, after all, in which we would all be winners and which everyone appears to believe in.

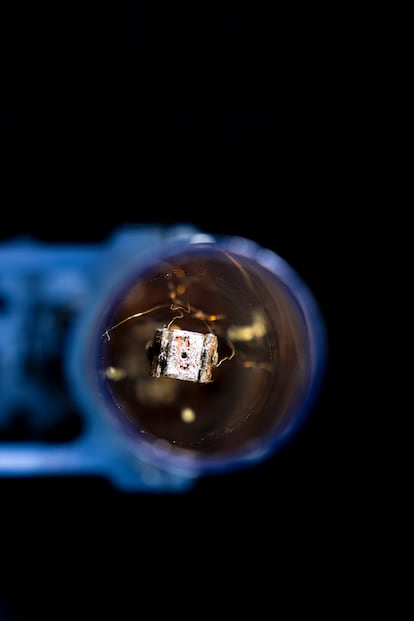

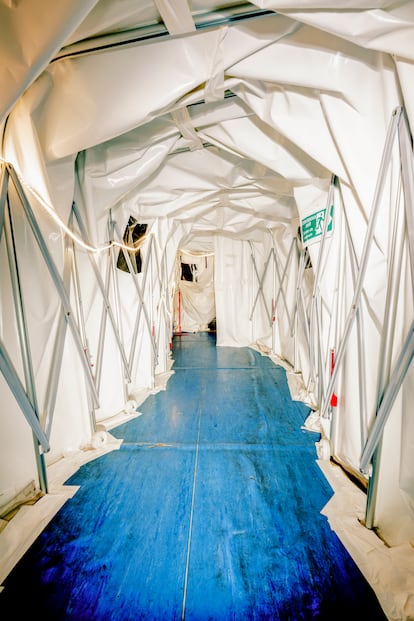
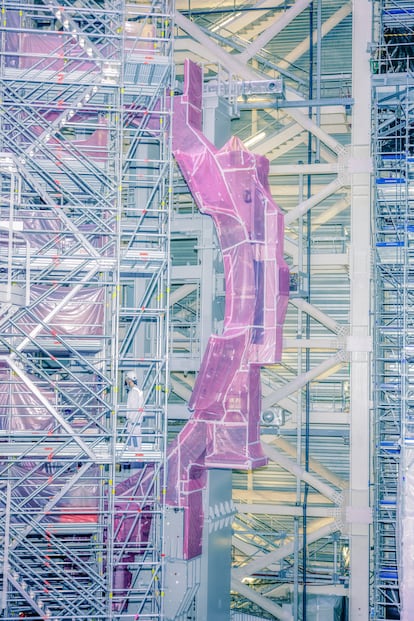

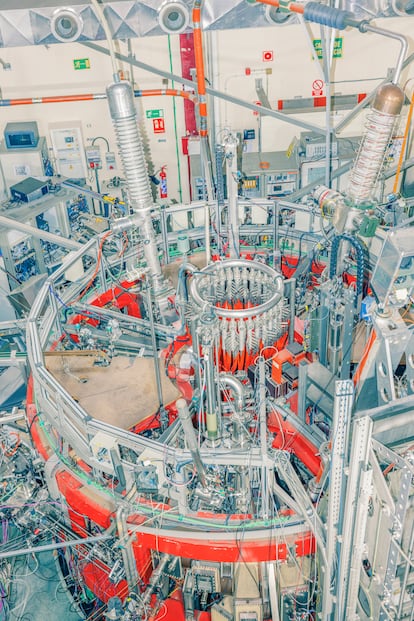
When asked for their predictions for the future, many of the scientists replied: “Fusion will come when we want it to come.” That is, when it is really needed and the resources are invested. The need for clean energy is more urgent than ever; the consequences of climate change are already being felt and it seems clear that more alternatives to fossil fuels are needed. By 2050, electricity demand is forecast to rise between 30% and 76% and renewables will not be able to meet all the demand.
What is still not clear is what reactor will win the race, says José Manuel Perlado, president of the Guillermo Velarde Nuclear Fusion Institute at the Polytechnic University of Madrid. “In these races, it is not necessarily the best who wins,” he says. “For now there are many ideas on offer, nobody can tell you exactly where the issue will go in the end and the big energy companies are not going to risk investing until it is clearer.”
If Livermore was a turning point on the road to nuclear fusion energy, the next, and surely definitive, will be the day when the major electricity companies start investing for real.
Credits
Sign up for our weekly newsletter to get more English-language news coverage from EL PAÍS USA Edition