Carla Marín, physicist: ‘We try to reproduce the conditions that existed at the origin of the universe’
The researcher, who was recently recognized by the Spanish Royal Society of Physics and the BBVA Foundation, talks about mysteries like dark matter and the lack of antimatter
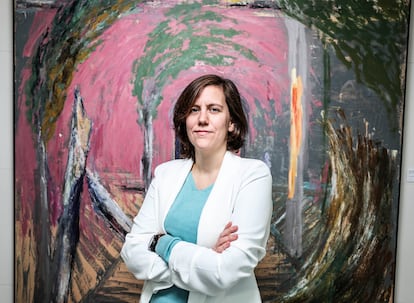
In 2012, the European Laboratory for Particle Physics (CERN) announced the discovery of the Higgs boson. This particle, which explains why matter has mass, confirmed predictions made in 1964 and is a fundamental piece of the Standard Model, the theory that explains the behavior of the particles that make up the world. The discovery was possible thanks to a huge infrastructure known as the Large Hadron Collider (LHC), a ring 27 kilometers (17 miles) in diameter built near Geneva, Switzerland, that cost approximately $9 billion. Inside, protons circulate directed by large magnets, colliding with each other and recreating situations that have not existed in nature since the Big Bang.
The extraordinary interest in fundamental physics that this milestone attracted has since faded, but the machine continues to work in search of information with which to understand what the cosmos is made of. Spanish physicist Carla Marín is one of the scientists who continue to test the limits of the Standard Model; Marín, a professor at the University of Barcelona, was recently awarded as a top young researcher in experimental physics by the Spanish Royal Society of Physics and the BBVA Foundation.
In Geneva, Marín collaborates on the LHCb detector, one of the four large experiments in which the LHC makes protons collide to test the limits of physics between the disintegrations that remain after the impact. “We specialize in studying b-type quarks, the heaviest we know,” says Marín. “We measure very precisely how they are created, how they associate with other quarks or how they disintegrate, because they are unstable particles, and compare that behavior with what the theory says, to see if at some point it breaks down. We look for new physics, indirectly,” she explains.
Question. Can you remind us what it is that you are doing at the LHC?
Answer. We accelerate protons at a very high speed, giving them a lot of energy, and when they reach their highest possible level of energy we make them collide. The fact of having so much energy is what produces these particles that have more mass. They don’t just appear out of thin air; we are transforming energy into matter.
Q. Have these particles that appear after the collisions ever existed in the universe?
A. We think so, but we don’t know. It is believed that at the beginning of the universe, when it was very energetic, very hot and very dense, it was concentrated in a very small space. The Big Bang Theory tells us that, at that moment, there was a state, a quark soup, with all these quarks that we know, the six quarks that the Standard Model predicts, which circulated freely due to the amount of energy that there was. We try to reproduce the conditions that existed at the origin of the universe.
Q. You have been awarded a Starting Grant from the European Research Council to carry out the CLIMB project. What are you going to look for?
A. We want to study the decays of a b quark, which is one of the most massive, to a d quark, which is one of the lightest, and two leptons. Why? In the Standard Model, this is possible, but it happens very rarely; once in every 100 million times a b quark decays. If there is another particle, another force that we do not know so far, that the standard model does not predict, but that exists in nature, it can affect these types of decays. The rarer this decay is in the Standard Model, the more sensitive it will be to any effect, no matter how small, that falls outside the model and that could indicate that there is a new particle or a new force interacting with the b quarks.
Q. Einstein looked for a unifying theory that could explain the entire universe. Is it reasonable to continue to search for this type of theory, or does reality work differently?
A. We don’t really know. There are unified theories with which we try to explain the forces that exist in nature from a common origin, but they are not very fashionable right now. The problem is verifying if what they predict happens in nature or not. We would need much more energy than we can now create in experiments to see its effects. There might be a point where the forces we study behave a little differently, but from what we know now, this will almost certainly happen at a very high energy, which we don’t have access to right now.
There has been talk — in a somewhat science fiction idea — of creating an accelerator around the Earth. You would need something at this scale, and with the technology we have it is not feasible. What we are doing is looking for new ways to accelerate particles, beyond those we currently use with superconducting magnets. Plasmas and other technologies are being studied to achieve greater accelerations in less space, but we do not know how long it may take to develop these new technologies.
Q. One of the particles that are sought, but not found, is the one that would make up dark matter.
A. We have very clear evidence, especially on the scale of the universe, when we observe galaxies, that we need matter that we don’t know in order to explain the gravitational effects that we see. We’ve looked in many places and haven’t found anything so far; sometimes I wonder if we’re looking for dark matter in the wrong places, if we have to turn the problem around. Variations on the theory of gravity have been proposed, for example, but they don’t seem very promising. We are looking in all possible places, in very small masses that interact a lot and very large masses that interact very little, but we haven’t seen anything anywhere, so I wonder if we are not misapplying the knowledge we have.
Q. It feels like there were much more spectacular advances at the end of the 19th century and the beginning of the 20th, with the atomic model, the quantum theory, relativity or the Big Bang. Is progress slower now?
A. I think it’s true, progress is a little slower, but I think the difference is precisely that at the time that you mention we surpassed the technical barrier to be able to see, for example, quarks. You were able to see that level of small things that is related to the energy you need to put into your system. Since then, we have moved forward, but we have done so in a very linear way. We have been able to create and observe the Higgs boson in our collisions, but in the end we are talking about the same energy range. We are not talking about different orders of magnitude. We may have already seen everything there is to see in this range we’re in and need a leap, not just a linear advance in technology, to get to the next state. Without this technology, without being able to make this technological leap to have the new accelerators that I mentioned before, it will be very difficult for us to reach the next level.
Sign up for our weekly newsletter to get more English-language news coverage from EL PAÍS USA Edition
Tu suscripción se está usando en otro dispositivo
¿Quieres añadir otro usuario a tu suscripción?
Si continúas leyendo en este dispositivo, no se podrá leer en el otro.
FlechaTu suscripción se está usando en otro dispositivo y solo puedes acceder a EL PAÍS desde un dispositivo a la vez.
Si quieres compartir tu cuenta, cambia tu suscripción a la modalidad Premium, así podrás añadir otro usuario. Cada uno accederá con su propia cuenta de email, lo que os permitirá personalizar vuestra experiencia en EL PAÍS.
En el caso de no saber quién está usando tu cuenta, te recomendamos cambiar tu contraseña aquí.
Si decides continuar compartiendo tu cuenta, este mensaje se mostrará en tu dispositivo y en el de la otra persona que está usando tu cuenta de forma indefinida, afectando a tu experiencia de lectura. Puedes consultar aquí los términos y condiciones de la suscripción digital.