The project that seeks the origin of the universe under a mountain in the Pyrenees
At the Canfranc Underground Laboratory in northern Spain, a team of international scientists is building a machine to unravel the mysteries of dark matter and answer the question: Why are we here?
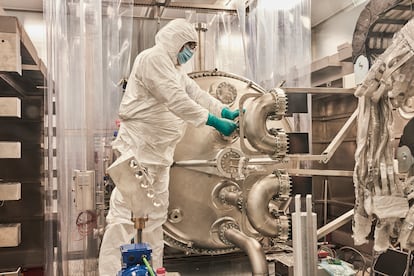
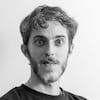
It was a good day to be underground. Canfranc, a village of 612 inhabitants in the Spanish Pyrenees, woke up looking gloomy, the color of its buildings dulled by dense, impenetrable clouds, with residents confined to their homes, almost no tourists on the streets, and a biting cold that cut the lips of anyone who ventured out. It was the kind of day that made one wonder, more than ever: why are we here? At the Canfranc Underground Laboratory (LSC), just a few miles from the French border, an international team of scientists is trying to find at least part of the answer to that question that has obsessed humanity since the beginning of time.
It’s 10:30 a.m. Carlos Peña, the director of the LSC, finishes his meeting in the research center’s offices— a gray building with straight lines that contrast with the mountains in the background — puts on a fluorescent yellow work coat, and gets into the van. On the way to the lab, Peña tries to explain the most important experiment underway. “The fundamental question is: why did nature choose matter over antimatter?” To understand the LSC’s contribution to the answer, a couple of things need to be explained first.
The known universe — stars, planets, flowers, and the air — is made of atoms, and these are composed of three fundamental particles: protons, neutrons and electrons. These three particles are matter, meaning they also have an opposite: their antiparticle. The electron’s antiparticle is the positron, which has a positive charge, as opposed to the electron’s negative charge. But there’s another particle that fascinates scientists: neutrinos. They are perhaps the most special of all: they exist in abundance in the universe, but barely interact with matter, have no negative or positive charge (unlike protons and electrons), and they pass through the Earth almost undetected.
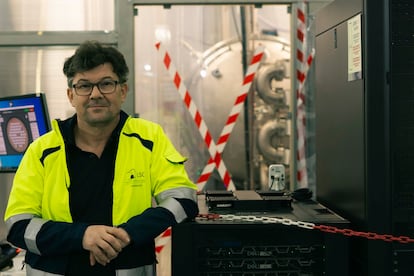
Their most interesting characteristic is that they are neutral, meaning they can be both matter and antimatter at the same time. Peña explains: “In the description of nature given to us by quantum mechanics, when one thing is indistinguishable from another, it is both at the same time. That’s why we believe the neutrino is both matter and antimatter.” Since the neutrino has no charge or internal structure, its antimatter version (the antineutrino) should be identical to it. This is essential to understanding the LSC experiment.
Peña enters the Somport tunnel, which connects Spain with France, and proceeds through an interior detour until reaching a large white door: the Canfranc Underground Laboratory. The size of an industrial warehouse, it was inaugurated in 2006, and there are only three similar laboratories in Europe, as well as in the United States, Canada, Korea, and Japan. The LSC is made up of several rooms, and in each of them different experiments are carried out: some try to detect wimps (a possible type of dark matter), others search for axions (possible dark matter particles), and a biology laboratory studies how low cosmic radiation affects living organisms.
Above the LSC, there is the Candanchú ski resort, 800 meters of land that are essential for the proper functioning of the detectors housed in the laboratory. Here, the ultra-fine particle detectors are almost isolated from the noise of the universe, the cosmic rays: high-energy particles coming from the Sun, supernovae and other galaxies. When these rays collide with the atmosphere, they generate a shower of secondary particles that pass through the surface. The mountain acts as a natural shield against these unwanted particles.
“If that cosmic noise were to reach here, it wouldn’t let us see the improbable phenomena we’re trying to detect here,” explains Peña. Neutrinos, however, pass through the mountain without difficulty to the gigantic detector installed at the LSC. It’s the largest room. It’s not cold. “It’s 18ºC [64ºF] here, compared to the 8ºC [46ºF] on the mountain out there,” says Peña.
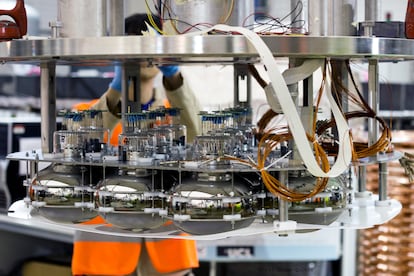
The site is as large as a warehouse in an industrial park. Two workers move around the space touching things, while Peña chats briefly with a third, a scientist who has come to give instructions and review the project’s progress. About 30 people work here on a daily basis, although the total team numbers nearly 300 researchers operating remotely from around the world.
In the main space, there is a glass-enclosed room that cannot be accessed, and inside, a small version of the machine they intend to use to capture this phenomenon, which has only been theorized on and would allow them to take a step toward explaining the origin of the universe — or rather, our existence in it. The experiment is part of the NEXT project, an international collaboration led from Spain that already includes more than 130 researchers.
Peña returns to neutrinos. Quantum physics has calculated — “we believe,” says Peña — that the neutrino is both matter and antimatter, “and that only when we detect it does it become one of the two. Before we do, it is both at the same time.” And what use is this to explain the origin of the universe? The final answer is near.
But there’s a small problem: the universe should be pure emptiness. The laws of physics dictate that the same amount of matter and antimatter were created in the Big Bang. This wasn’t the case because we exist, and therein lies one of the great mysteries that quantum physics is trying to resolve. When a particle of matter (an electron) joins with its antimatter particle (a positron), they annihilate each other, leaving only energy (i.e., light). If everything had been perfectly symmetrical, the universe wouldn’t exist. Mathematics dictates there shouldn’t be galaxies, stars, planets, or people. Only light. But here we are. “Most of the universe is light; we’re only three parts out of every 10 billion,” Peña explains.
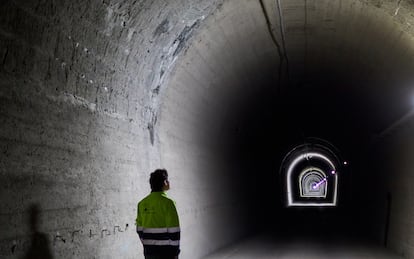
What does that mean? That something broke that perfect symmetry, and an excess of matter remained. “Matter won by a little.” Physicists don’t know why, and they believe the neutrino holds the answer. “That kind of spy, which can talk to matter and antimatter, meant the annihilation wasn’t perfect, and matter ultimately won,” he says, fascinated despite the number of times he’s explained this to visitors.
“What we’re trying to demonstrate here is that the neutrino is both matter and antimatter,” he says, standing in front of the detector they’ve been developing for years. We hope it’s the first version before the final version, which will be able to detect this interaction (two neutrinos annihilating each other) once a year. They already have the container, a tank almost five meters high.
They’ll use the technology currently installed in a smaller detector (about two meters long) that they’ve installed. Why can’t that interaction be detected with that one? “Because, according to our calculations, it could only be detected once every hundred years with a detector of this size,” Peña explains.
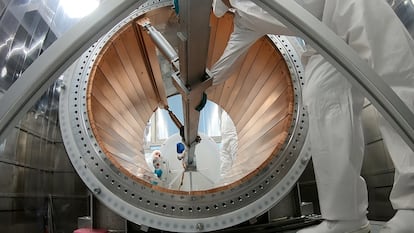
The next vital experiment for physics is being developed in Japan and attempts to demonstrate the other theorized part of this explanation for the origin of the universe: that when two neutrinos annihilate each other, a remnant of matter remains. That experiment will have results by 2030. With the Canfranc experiment, we will have to wait until 2035 to find out if neutrinos can, in fact, be matter and antimatter. “It’s an essential answer. It would allow us to know where we come from, where the pieces that make us come from. We would know the mechanism by which protons, neutrons, and electrons won,” Peña says.
The exit from the laboratory is different from the entrance. Peña gets into the van and says, “Let’s go out through the railway tunnel.” This is the origin of the LSC: a tunnel, built in 1928 to connect Spain and France, which fell into disuse in 1970 after the collapse of a bridge on the French side. The physicist Ángel Morales, a professor at the University of Zaragoza, and his team, armed with rudimentary detectors and a great deal of ingenuity, arrived at the tunnel in a van around 1985. They fitted the vehicle with railway wheels so it could move over the railway tracks. They transported a small mobile laboratory and traveled through the tunnel, analyzing its conditions and measuring radiation levels.
That improvised experiment confirmed what they suspected: the enormous mass of rock above their heads blocked cosmic rays, providing an ideal environment for detecting extremely rare particles, such as neutrinos, and possible traces of dark matter. That first trip, carried out with barely any resources, was the seed of what, decades later, would become one of the most advanced scientific infrastructures in Europe.
This article was originally published as part of Tendencias (Trends), a project by EL PAÍS, with which the newspaper aims to open an ongoing conversation about the major future challenges facing our society. The initiative is sponsored by Abertis, Enagás, EY, GroupM, Iberdrola, Iberia, Mapfre, the Organization of Ibero-American States (OEI), Redeia, Santander, and strategic partner Oliver Wyman.
Sign up for our weekly newsletter to get more English-language news coverage from EL PAÍS USA Edition
Tu suscripción se está usando en otro dispositivo
¿Quieres añadir otro usuario a tu suscripción?
Si continúas leyendo en este dispositivo, no se podrá leer en el otro.
FlechaTu suscripción se está usando en otro dispositivo y solo puedes acceder a EL PAÍS desde un dispositivo a la vez.
Si quieres compartir tu cuenta, cambia tu suscripción a la modalidad Premium, así podrás añadir otro usuario. Cada uno accederá con su propia cuenta de email, lo que os permitirá personalizar vuestra experiencia en EL PAÍS.
¿Tienes una suscripción de empresa? Accede aquí para contratar más cuentas.
En el caso de no saber quién está usando tu cuenta, te recomendamos cambiar tu contraseña aquí.
Si decides continuar compartiendo tu cuenta, este mensaje se mostrará en tu dispositivo y en el de la otra persona que está usando tu cuenta de forma indefinida, afectando a tu experiencia de lectura. Puedes consultar aquí los términos y condiciones de la suscripción digital.