A prodigious transformation: A star has been born from the cold cosmic void
Few transformative processes in nature are as extreme as the formation of a star. Those that give light and sustain life on at least one planet were once little more than extremely cold and barely visible clouds of gas
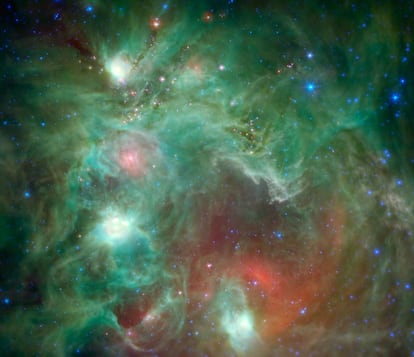
With the stars — like the Universe — as soon as we began to understand how they work, we learn that they haven’t always been there.
Our own Sun provides evidence of this perishable nature. It consumes hydrogen in thermonuclear reactions at a rate of 100 million tons per second, which is why it shines. However, while it has a large amount of mass, it’s not infinite. The conclusion is obvious: the Sun hasn’t always been shining, nor will it shine forever.
Stars, therefore, have a beginning, just as we can say that they experience something that resembles an end. Starting from what we know as the interstellar medium, the formation process of a star involves a prodigious transformation: tenuous and very cold material — around 263 degrees below zero — transforms into an incandescent fireball, with temperatures that will reach millions of degrees inside. Meanwhile, its density will increase exponentially, by many zeros.
The first thing we need to understand is that this is a process that takes time. Not as long as a star shines, of course, but still quite a bit (even on a cosmic scale). It’s by no means an instantaneous process. If the right conditions are met, in tens of millions of years, the cosmos can form a star.
The mechanism is chaotic and full of uncertainties. Hundreds and thousands of stars form at the same time in dusty gaseous envelopes streaked with bubbles. They’re spread and held together by the solid particles we call “interstellar dust.” For this process to occur, it takes — as has already been mentioned — time. But, above all, it’s necessary to transform the conditions of the environment several times, so that it goes from temperate and almost empty, to very cold and dense. Over time, with more density, it can continue to collapse, due to the effect of gravity.
Threadlike and tenuous low-density material ends up fragmenting into thousands of clumps that will gradually give rise to the densest structures in the cosmos. Some are hundreds of times the mass of our star and come mostly in pairs — in so-called “binary systems” — and others (the majority) appear in the form of smaller stars, almost like the Sun, only a little less massive.
We don’t fully know the process behind how massive stars are formed. These are the ones that have, say, more than 20 times the mass of the Sun. It’s not yet clear if they form as a result of the monolithic collapse of a very large cloud or if, on the contrary, they become large as a result of smaller stars being added together. The detection of gravitational waves or new discoveries — such as the black hole 33 times the mass of the Sun recently discovered by Gaia, a European Space Agency observatory mission — show that we’re still not clear about all the details.
The fascinating thing is that the birth of the largest stars is connected to that of the smallest stars, as well as to the formation of stars throughout an entire galaxy. Massive stars — through their winds and supernova explosions — inject enormous amounts of energy into the interstellar medium, thus reducing the rate of formation of other stars. Without massive stars, our Milky Way would exhaust its gas in a huge, brief burst of formation. However, thanks to them, it has continued to slowly form stars for billions of years.
For stars like the Sun, on the other hand, we understand the procedure in general terms, because it’s easier to see this with telescopes. We know that clouds of a certain diameter — around three to 30 light years — transform into smaller and more intricate clouds that can stretch out to 10,000 times the distance between the Earth and the Sun. This process occurs in very cold environments (perhaps with some turbulence) and vortices where the material is concentrated, becoming more and more dense. That’s where the mass falls.
As the material that comes from afar approaches the center — the point of collapse — or the edge, it has a certain rotation speed associated with it that increases. It’s precisely this conservation of angular momentum that gives rise to the formation of a disk that fulfills two functions: on the one hand, it feeds the star with material so that it can continue growing and, on the other, it’s that same structure associated with the formation of the star itself that allows planets to form.
What makes the spark ignite? Well, the required condition for us to consider them to be stars is that, at a particular moment, they’re capable of igniting nuclear fusion reactions within them. This is when the protostar becomes a star. Of course, some fail: the very small ones that are neither one nor the other, neither a star nor a planet. These are known as “brown dwarfs.”
With time and gravity, stars will explode as supernovas, collapsing as neutron stars, white dwarfs and even black holes. But before that — and as incredible as it may seem — to form the hottest structures, the Universe simply requires the cold.
Sign up for our weekly newsletter to get more English-language news coverage from EL PAÍS USA Edition
Tu suscripción se está usando en otro dispositivo
¿Quieres añadir otro usuario a tu suscripción?
Si continúas leyendo en este dispositivo, no se podrá leer en el otro.
FlechaTu suscripción se está usando en otro dispositivo y solo puedes acceder a EL PAÍS desde un dispositivo a la vez.
Si quieres compartir tu cuenta, cambia tu suscripción a la modalidad Premium, así podrás añadir otro usuario. Cada uno accederá con su propia cuenta de email, lo que os permitirá personalizar vuestra experiencia en EL PAÍS.
En el caso de no saber quién está usando tu cuenta, te recomendamos cambiar tu contraseña aquí.
Si decides continuar compartiendo tu cuenta, este mensaje se mostrará en tu dispositivo y en el de la otra persona que está usando tu cuenta de forma indefinida, afectando a tu experiencia de lectura. Puedes consultar aquí los términos y condiciones de la suscripción digital.